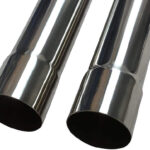
Food and Beverage Pipe Bending
January 4, 2024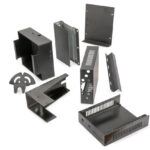
One-Stop Sheet Metal Fabrication
February 13, 2024Basic Knowledge of Metal Plastic Deformation
Sheet metal, straightening, bending, rolling, stamping, and other processes in cold working utilize the plastic deformation of metals at normal or high temperatures to achieve the desired shapes. Therefore, metal plastic deformation is the foundation of metal forming.
(1) Cold Plastic Deformation of Metals
When metals are subjected to external forces in a cold state, their shapes and dimensions undergo changes, which can be either elastic or plastic. Elastic deformation refers to the ability of metals to recover their original shape and dimensions once the external force is removed. Conversely, plastic deformation occurs when the shape change is permanent.
Metals are composed of many grains, and plastic deformation of metals is a result of relative slip between internal grains. Thus, the most basic mode of plastic deformation in metals is slip. Since metals are mostly polycrystalline, consisting of many small grains with different orientations, the grains that are most favorable for slip will initiate slip first and gradually propagate to other grains. Therefore, the resistance to plastic deformation is greater in polycrystalline metals with finer grains and more grain boundaries.
During cold plastic deformation of metals, distortion and deformation occur in the lattice near the slip plane, causing grain fracture in the slip region. This hinders further slip and leads to an increase in strength and hardness, as well as a decrease in plasticity and toughness. This change in mechanical properties due to cold working is known as work hardening or strain hardening.
Work hardening is an important property of metals, as it not only increases the energy required for further deformation but also makes forming more difficult. Additionally, the reduced plasticity and toughness resulting from work hardening may lead to cracking and fracture during forming. To prevent such phenomena, some cold-worked parts need to undergo annealing heat treatment. Therefore, work hardening has significant practical significance. However, work hardening also has its useful side, as it can increase the strength of certain components through strain hardening.
Another consequence of cold plastic deformation of metals is the generation of internal stress. The presence of internal stress weakens the strength of metals, and when released, it can cause deformation of the workpiece.
(2) Recrystallization of Metals
When metals that have undergone cold plastic deformation are heated to higher temperatures, the increased atomic activity leads to the rearrangement of distorted and fractured grain atoms, resulting in the formation of new nuclei and continuous growth of these nuclei until the cold-worked structure of the metal completely disappears. This process is known as recrystallization.
After recrystallization, the strength and hardness of metals significantly decrease, while their plasticity and toughness greatly improve. Internal stress is completely eliminated, returning the metal to its original state.
(3) Thermal Plastic Deformation of Metals
Pressure processing of metals above the recrystallization temperature eliminates the work hardening caused by plastic deformation, which is commonly referred to as hot working.
During thermal plastic deformation, two opposite processes occur within the metal: work hardening and recrystallization softening. These processes occur simultaneously during deformation. When the degree of deformation is large, but the heating temperature is low, the strengthening caused by deformation dominates, resulting in an increase in strength, hardness, and a decrease in plasticity and toughness. The lattice distortion of the metal cannot be recovered, and the resistance to deformation becomes increasingly significant, potentially causing metal fracture. Conversely, when the degree of deformation is small and the heating temperature is high, recrystallization and grain growth dominate, resulting in coarsening of the grains and deterioration of the metal’s properties. Therefore, it is necessary to balance the heating temperature and degree of deformation during hot working.
(4) Temperature Range for Hot Working of Steel
The temperature at the beginning of forging steel is called the initial forging temperature, while the temperature at the end of forging is called the final forging temperature. The range between the initial and final forging temperatures is referred to as the forging temperature range or the temperature range for hot working. Higher heating temperatures yield better plasticity and lower resistance to deformation, making it easier to form the metal. However, excessively high temperatures can lead to overheating or burning of the steel, causing severe oxidation and decarburization.
Overheating occurs due to excessively high heating temperatures or prolonged holding times. It causes coarsening of the austenite grains, resulting in decreased mechanical properties, especially plasticity. Overburning occurs when the steel is heated close to its melting point, causing oxygen to infiltrate along grain boundaries, leading to oxidation and embrittlement of the grain boundaries, resulting in reduced plasticity and rendering the steel unable to be formed.
The maximum heating temperature (initial forging temperature) of steel is usually 200°C lower than the solidus line. The final forging temperature should ensure sufficient plasticity and facilitate the formation of a recrystallized structure. For hypoeutectoid steels, the final forging temperature can be 15-30°C above AC3. However, for steels with carbon mass fractions below 0.3%, the final forging temperature can be lower than AC3 due to sufficient plasticity. For hypereutectoid steels, it can be 50-100°C above the A1 line.
Some hot forming processes are carried out at relatively low temperatures, such as hot stamping and flanging of elbows and vessel nozzles at around 700-750°C, and mid-temperature rolling and straightening of cylindrical sections at approximately 650°C. When processing these parts, the embrittlement temperature range of the steel should be considered to avoid adverse effects on their performance.
The color of steel changes during heating, which can be used as a rough indicator of the heating temperature.